Your First Choice Program?
We continue looking at some admissions data for some Ontario engineering programs, based on the CUDO public database. As a bit of background, applicants to Ontario universities all apply through a central system (OUAC) shared by the universities (which is where this data comes from, I assume). In this system, an applicant can rank their preferred choice of program and university. Presumably, applicants rank as first choice the university they most desire to attend. Let’s see from the data where people tend to want to go, and how many end up there after the admissions process is all done.
Continue readingEngineering Tuition Trends
Like some other professional programs, the tuition for engineering tends to be more expensive than of many other degrees. It’s useful to compare tuition across some of the Ontario universities, once again using the CUDO data to see if there are any significant differences. We can also look at the trends over a few years to see how much more expensive it’s been getting. For context before we get into the engineering fees, according to CUDO in 2022 a typical “Arts & Science” program fees is around $7,500 for the first year (including ancillary fees). So on to the engineering fees.
Continue readingEntrance Average Breakdowns
In a recent couple of posts we’ve looked at the overall entrance averages for some Ontario engineering programs, and the specific proportion of admitted students that had a 95%+ average. Let’s look at the full breakdown of the proportions of admitted students with averages of 75-79, 80-84, etc. This data gets a bit too messy to plot across several years, so I’ll just look at 2021, the most recent year available on the CUDO data website.
Continue readingAdmitted With a 95%+ Average
The previous post showed the overall averages of students admitted to engineering programs (based on CUDO and Ontario school data). Since CUDO provides the data broken down into grade range bins, let’s look at one in particular. This is the bin for admitted students with an admission average of 95% or higher. The graph below shows the proportion (%) of admitted engineering students that had a final admission average greater than or equal to 95%, from 2017 to 2021 (the last available year)
Continue readingEntrance Average Trends
Everyone is always excited, concerned, interested and/or complaining about entrance averages, whether your a potential applicant, parent, faculty member, secondary school teacher, accreditation board, etc. So let’s have a look at how Ontario engineering school entrance averages have faired over the past few years. As usual, the data is from CUDO and in this case it is only available up to the 2021 entrance.
Continue readingWomen in Engineering Trends
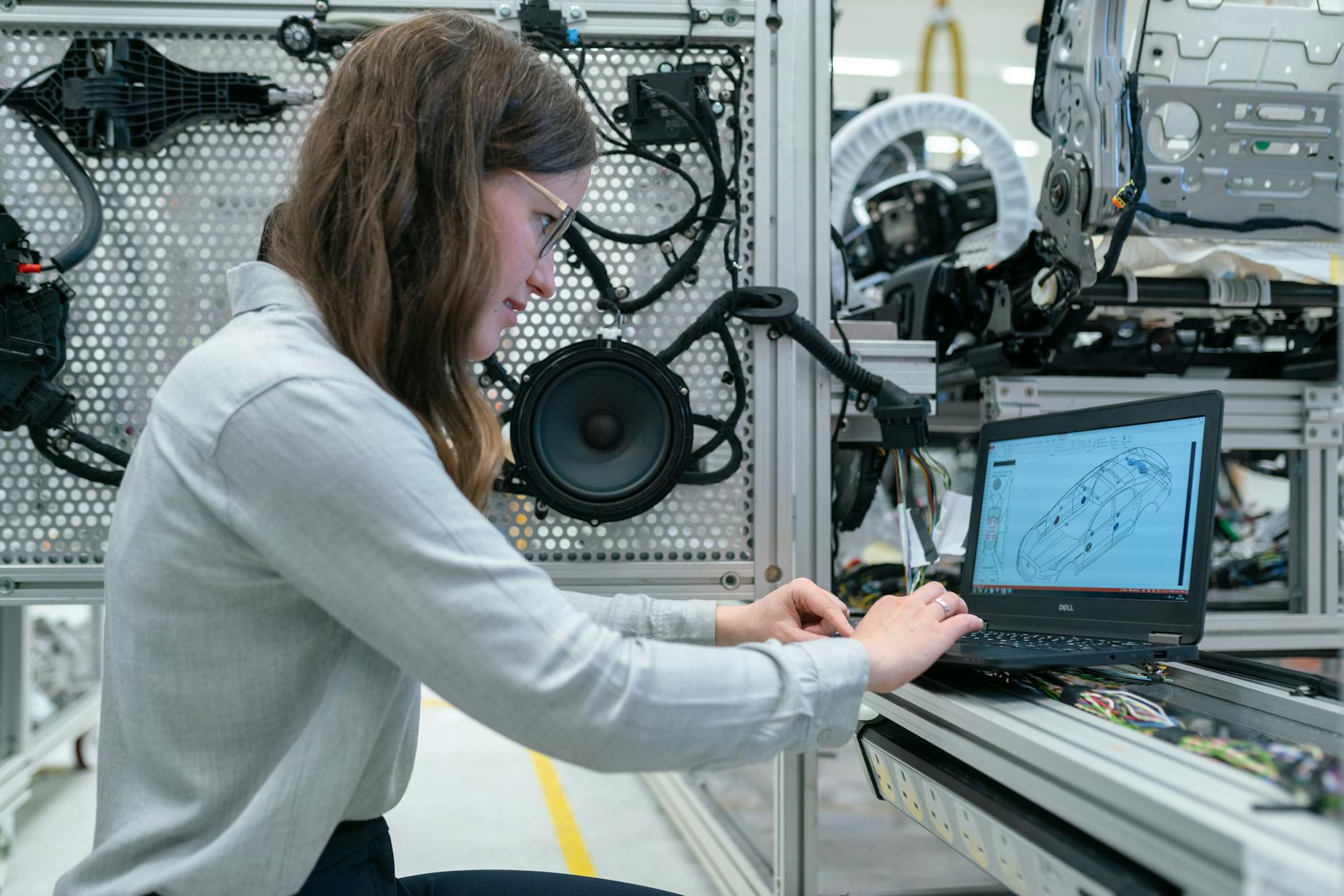
The Common University Data Ontario (CUDO) website has lots of accessible information about Ontario universities, and it’s been quite a while since I looked at it. Since there’s nothing more fun for engineers than compiling and looking at data, I’ll post some now and then. Today, let’s start by looking at female, full-time enrollment in some Ontario engineering programs, and how it has trended over the past few years.
Continue readingKilling Germs on Escalator Handrails
An example of some of the research we do in engineering. I collaborated on a small project to test how to apply antimicrobial copper coatings to the rubbery surfaces of escalator handrail materials, using a technology called “cold spray”. It’s essentially a high velocity shot of copper particles onto the surface, and my mechanical engineering colleague Prof. Jahed led the project with his expertise in this area. Getting metallic materials to permanently stick onto rubbery materials is no easy task, but this approach seemed to work pretty well. There are a lot of microscopic technical issues that need to be understood.
My other colleague, Prof. Marc Aucoin from Chemical Engineering and I helped assess the anti-viral and antibacterial efficacy of the material after coating. Basically we showed that it was pretty good and various viruses and bacteria died off at very respectable levels (over 99.99%) when exposed to the surfaces.
This was a nice collaborative project involving professors, students and other researchers from mechanical and chemical engineering, and a combination of materials science, virology and microbiology. The resulting article was published last year in the Journal of Thermal Spray Technology, and was recently picked as one of 6 “Editors Choice” articles in 2023 in that journal. Anyone can now read it without a subscription, at this link. I’m not sure where this might lead next, but it’s a small step forward in potentially reducing disease transmission in public areas.
Engineering Disasters
In posts on accreditation of engineering programs, I mentioned that ethics, professionalism, health & safety, and environment are all expected outcomes that graduating students should have some familiarity with. When I was a student (a long long time ago) these topics weren’t emphasized much, but thankfully they are now. In courses I have taught, I try to inject some examples of technical areas where special attention is required to minimize risks to health, safety and the environment.
It’s commonly known that physicians have ethical responsibilities to not harm their patients. A bad or incompetent physician might harm patients one at a time, typically, but bad or incompetent engineers and other technical people can harm dozens or even thousands of people all at once. Here are a few examples that come to mind, some of which I’ve used as case studies in courses. These incidents are often due to a series of issues and are not usually caused by a specific engineer or other person. But they illustrate why we need to emphasize how to recognize and prevent these sorts of things in engineering education.
Imperial Sugar Refinery Explosion: In 2008, a dust explosion at a sugar refinery in Georgia killed 14 and injured 36. Poor design, maintenance, hazard identification and risk mitigation.
Hyatt Regency Walkway Collapse: In 1981, overhead walkways at a hotel in Kansas City collapse, killing 114 and injuring 216 due to structural overload. The original (adequate) design had been modified during construction, but without proper design change management and review. Something vaguely similar happened to Terminal 2E at the Charles de Gaulle airport in Paris in 2004.
Piper Alpha Oil Platform Explosion: In 1988 the North Sea oil platform had an explosion and fire that essentially melted it into the sea, killing 167 with 61 escaping. There were numerous failures that contributed to it, but they include poor design and management of change, inadequate hazard recognition and mitigation, poor safety management system, and poor training.
Bhopal Gas Release: In 1984 a chemical plant in India had a toxic gas release of methyl isocyanate leading to 3,787 deaths and 574,366 injuries. Estimates vary, but the numbers are staggering no matter what the exact numbers. Again, there is a host of issues but they include: poor maintenance on multiple pieces of equipment and instrumentation, inadequate safety system design, poor emergency response systems, poor management of change. This event stunned the chemical industry and led to major increases in attention paid to “process safety“, including in chemical engineering education and program accreditation.
Texas City Refinery Explosion: In 2005 a flammable vapour cloud release lead to an explosion that killed 15 and injured 180. As usual, multiple factors at play including: poor design and maintenance, poor safety culture in the company, overloaded staff, poor hazard identification and risk mitigation, lack of investment.
So those are a few examples, mainly from the chemical industry because that’s what I’m more familiar with. There is a whole bunch more posted on the U.S. Chemical Safety Board website (a good resource for teaching materials). There are probably lots of others from various sectors that I could look for, including the obvious and infamous Chernobyl nuclear disaster. If anyone has interesting other examples feel free to post some information in the comments.
Accreditation Requirements: The Process
Previously in this series, I’ve summarized the why and what (content & outcomes) for engineering program accreditation. Let’s finish up with how it all works; what does an engineering program have to do to be accredited according the the criteria discussed previously?
First of all, the frequency. Engineering programs are accredited for at most 6 years (sometimes less if there are some concerns raised by the Canadian Engineering Accreditation Board, CEAB). So every 6 years the cycle described below is repeated for every engineering program in Canada (although not all in the same year!). For new engineering programs the cycle doesn’t start until the first students are graduating.
- The institution requests CEAB to assess or re-assess their engineering programs. Often, they try to do all the programs at the same time to save on time and effort.
- A visiting team is put together for each program, consisting of professional engineers familiar with that discipline. Typically there is a mix of faculty from other universities and people from industry.
- The institution puts together a tremendous pile of documentation for each program, which is sent to the visiting teams. This documentation provides evidence in support of each program’s content and outcomes described in the previous posts. The materials often include:
- Overall descriptions of each program, the structure, required and elective courses
- Descriptions of how admissions to the programs are handled (I had to deal with this in the past)
- Descriptions of facilities, student supports, etc. at the program level and higher.
- Financials for the programs, including investments in facilities and student support.
- Details on faculty, who teaches what courses, and whether they are licensed engineers.
- Details on each course in the program, its content, who teaches it, the course learning outcomes, how the outcomes are evaluated, typical class averages, and typical failure rates.
- Evidence for how the program meets the content criteria
- Evidence for how the program meets the expected accreditation outcomes
- Evidence and results for how the program continuously gathers feedback from graduates and industry to help implement continuous improvement of the program.
- The visiting teams review the documentation in advance, and then spend about 3 days at the institution to review the programs, facilities, personnel, in person. This will typically include:
- Interviews with students in each program
- Interviews with faculty teaching each program
- Interviews with the Dean and various other administrators for programs and the university
- Tours of the teaching facilities, labs, student workspaces, etc.
- Examination of teaching materials, such as course syllabi, textbooks, course notes, examples of student work such as assignments, reports and exams (both good and bad, but anonymous), projects completed by students, student transcripts (anonymous).
- After the visit, the teams compile reports which are sent to the CEAB for final decisions about whether accreditation will be granted, and/or for how long (i.e. 6 years, or less). The institution gets a copy of the reports and can respond to any inaccuracies in the report, or any weaknesses highlighted by the teams. These responses are also considered by CEAB before their final decision.
Ideally, the program gets a 6 year accreditation if there are no major concerns raised by CEAB. Then, after 6 (or whatever) years, the whole cycle starts again. At Waterloo, it’s an ongoing process during the 6 year period to continuously gather information that will be needed for the next accreditation cycle, so it never really ends. For example, when I teach a course I have to map the course content to the expected accreditation outcomes.
So, accreditation is a huge undertaking requiring a lot of documentation, information-gathering, planning, and visitor scheduling. This involves a lot of faculty and staff hours, and there are staff positions in Engineering that are largely dedicated to this process. I’ve never seen an estimate for how much this costs the Engineering programs, but I imagine it’s not cheap! Another factor that leads to engineering being a more expensive program to deliver, and so having higher tuition rates for students.
Accreditation Requirements: Outcomes
In this series I’ve covered why accreditation of engineering programs is important, and what the programs should contain. However, it’s not enough to just have the right program content. The programs have to also achieve a long list of “outcomes”, meaning that students graduating from the programs should have the following attributes:
- Engineering knowledge base: competence in math, sciences, engineering fundamentals, and discipline-specific knowledge.
- Problem analysis: ability to use the knowledge to solve engineering problems and make substantiated conclusions.
- Investigation: ability to approach complex problems using experiments, data analysis, and synthesis of information.
- Design: ability to meet goals and make decisions for complex, iterative and open-ended problems using all the tools available, including health & safety, sustainability, economic and other constraints.
- Use of engineering tools: ability to create, select or extend techniques and methods to solve problems, while understanding the limitations of these tools. This is often software & calculational tools, but can include other stuff.
- Individual and team work: ability to work as a member and leader in teams, preferably multi-disciplinary.
- Communication skills: ability to communicate complex engineering concepts to other engineers and the broader society. Includes reading, writing, speaking, listening, comprehending and writing effective reports & design documentation.
- Professionalism: understanding the roles & responsibilities of professional engineers, especially in protecting the public.
- Impact of engineering on society and the environment: understanding the interactions between engineering and societal effects such as economics, health & safety, legal, cultural, sustainability, environmental.
- Ethics and equity: ability to apply professional ethics, accountability and equity.
- Economics and project management: incorporating economics and business practices, including project, risk and change management, and knowing the limitations.
- Life-long learning: ability to identify and address personal educational needs to maintain competence in the field.
So everyone graduating from an engineering program should have achieved these attributes at some reasonable level, recognizing that they are just beginning a career and will continue to develop skills. In the next post, we’ll look at the actual accreditation process and how programs have to demonstrate that they have the right content and that their graduates have all the required attributes.